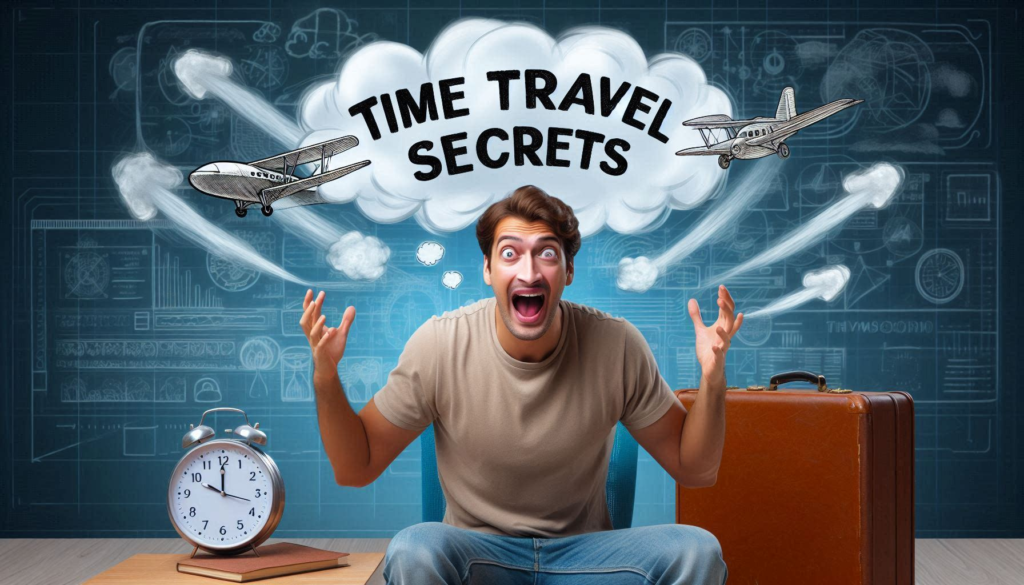
Introduction
The concept of time travel has long captured our imaginations, from the whimsical adventures in H.G. Wells’ “The Time Machine” to the intricate timelines of modern sci-fi blockbusters like “Interstellar” and “Avengers: Endgame.” But time travel isn’t just a staple of popular culture; it’s a fascinating and serious subject of scientific inquiry.
Physicists and theorists have been delving into the mysteries of time for decades, exploring how it might be possible to move through time in ways that challenge our everyday experiences. Recent advances in physics, particularly in the fields of relativity and quantum mechanics, have brought us closer than ever to understanding how time travel might work. These discoveries have not only expanded our knowledge but also challenged our perceptions of time.
In this blog, we’ll explore seven mind-blowing discoveries that provide interesting insights about the potential of time travel. From the fundamental principles laid down by Einstein’s theory of relativity to the mind-blowing implications of quantum entanglement and time crystals, each of these breakthroughs opens up new possibilities of what can be achieved.
Whether you are a science enthusiast, a fan of fiction, or just curious about the nature of time, these discoveries will provide a deeper understanding of how time travel could become a reality someday. So, get ready as we embark on a journey through cutting-edge science that could unravel the mysteries of time travel.
1.Einstein's Theory of Relativity: The Foundation of Time Travel:
Albert Einstein’s theory of relativity transformed our understanding of space, time, and gravity. It consists of two parts: special relativity and general relativity, both of which have profound implications on the concept of time travel.
Special Relativity: Time Dilation and the Speed of Light
Special relativity, introduced by Einstein in 1905, fundamentally changed the way we understand time and space. The two major principles of special relativity are:
- The laws of physics are the same for all observers, regardless of their relative speed.
- The speed of light in a vacuum is constant and will always be the same for all observers, regardless of their relative speed.
One of the most surprising results of special relativity is time dilation. According to this theory, time does not flow at the same rate for everyone; instead, it varies depending on the relative speed between observers.
Time Dilation Explained
Imagine two people: one on Earth and the other traveling in a spacecraft at a significant fraction of the speed of light. To the person on Earth, the clock on the spacecraft appears to run more slowly. This is because, at high speeds, time essentially “speeds up.” If the spacecraft travels close to the speed of light and then returns to Earth, the astronaut may find that only a few years have passed for them, while decades or centuries have passed on Earth. This phenomenon has been confirmed experimentally, such as in tests with precise atomic clocks placed on high-speed aircraft and satellites.
General Relativity: Gravitation and the Distortion of Space-Time
In 1915, Einstein expanded his theory to include the effects of gravity, leading to the development of general relativity. This theory describes gravity not as a force, as Newtonian physics does, but as a curvature of space-time caused by mass and energy.
Space-time and gravity
In general relativity, massive bodies such as planets and stars distort the spacetime continuum around them. This curvature affects the paths of objects and even light, causing them to follow curved paths. An important implication of this curvature is gravitational time dilation: the stronger the gravitational field (or the closer you are to a massive object), the slower time will pass.
For example, if you spend time near a massive object such as a black hole, time will pass much more slowly for you than for someone far away from the black hole. This effect has been observed with satellites orbiting Earth, where the clocks on GPS satellites run slightly faster than clocks on the ground because of the weaker gravitational field in orbit.
Relativity and the Possibility of Time Travel
Einstein’s theories of special and general relativity provide the theoretical framework for several time travel concepts:
- Time dilation: As described, traveling at relativistic speeds could allow forward time travel, where time passes more slowly for the traveler than for those left behind.
- Wormholes: These are hypothetical bridges in space-time that could potentially connect distant points in space and time, allowing instantaneous travel between them. If wormholes exist and can be stabilized, they could allow travel between different times.
- Gravitational time dilation: Harnessing strong gravitational fields could enable future technologies to manipulate the flow of time, potentially opening the door to time travel.
Practical considerations and challenges
While the theoretical foundations of time travel are solid, practical implementation poses immense challenges. Achieving the speeds needed for significant time dilation, creating or finding stable wormholes, and dealing with the enormous gravitational forces needed for gravitational time dilation are all beyond our current technological capabilities. Additionally, time travel raises many paradoxes and questions, such as the grandfather paradox, that are still unresolved.
2. Wormholes: Theoretical Passageways Through Time
Wormholes are one of the most intriguing concepts in theoretical physics. These hypothetical structures, also known as Einstein-Rosen bridges, are essentially tunnels in the fabric of space-time that can create shortcuts between distant regions of the universe. Let’s delve deeper into the fascinating world of wormholes and explore their potential implications for time travel.
Origin of Wormholes
The concept of wormholes originates from Einstein’s solutions to his field equations in his general theory of relativity. In 1935, Albert Einstein and physicist Nathan Rosen proposed the idea of bridges in space-time, which we now refer to as wormholes. These bridges theoretically connect two different points in space and time, allowing travel between them.
Structure of Wormholes
A wormhole has two mouths and a throat connecting them. Imagine the space-time continuum as a two-dimensional surface. If you fold this surface and create a tunnel between the two points, you have imagined a wormhole. The mouths of the wormhole may be located light-years apart in space or even in different periods.
Types of Wormholes
Two main types of wormholes are discussed in theoretical physics:
- Permeable wormholes: These wormholes can allow matter to pass between their two mouths. Theoretical physicist Kip Thorne and his colleagues have extensively studied the conditions necessary for a wormhole to be traversable by humans or spacecraft. For a wormhole to be stable and permeable, it would require the presence of exotic matter with negative energy density to prevent it from collapsing.
- Non-permeable wormholes: These, often referred to as Schwarzschild wormholes, exist as part of the solution to Einstein’s equations but collapse too quickly for anything to pass through them. They are not practical for travel because they close before anything can pass from one side to the other.
Wormholes and time travel
The idea of using wormholes for time travel is a fascinating possibility. If one mouth of a traversable wormhole could be accelerated too close to the speed of light and then accelerated back to its original position, time would pass differently for the two mouths due to time dilation. This means that if you enter the wormhole, you could potentially exit at a different time, making wormholes a potential mechanism for time travel.
Challenges and paradoxes
While wormholes offer exciting possibilities, they also come with significant challenges and paradoxes:
- Stability: Keeping a wormhole open and stable would require exotic matter with negative energy density, which has not been discovered or produced in the quantities needed.
- Causality violation: Time travel through wormholes gives rise to paradoxes such as the “grandfather paradox,” where a time traveler can potentially alter past events in ways that preclude their existence.
- Energy requirements: The energy required to create and maintain wormholes is beyond our current technological capabilities.
Current research and prospects
Although wormholes remain theoretical, they continue to be a subject of intense study in the field of theoretical physics. Researchers are exploring various aspects of quantum mechanics, general relativity, and exotic matter to understand the feasibility of wormholes better.
Recent advances in quantum physics and the study of black holes have provided new insights that may one day help us understand whether wormholes can exist and be used. While we are still far from practical applications, the study of wormholes continues to push the boundaries of our knowledge about the universe.
3.Cosmic Strings: Threads of Time:
Cosmic strings are fascinating hypothetical structures in the universe that emerge from theories about the early universe and the nature of space-time. These one-dimensional topographic defects may be remnants of phase transitions that occurred in the early universe, the way cracks form in ice when water freezes. Here’s an in-depth look at what cosmic strings are and their potential implications for time travel:
What are cosmic strings?
Cosmic strings are incredibly thin, yet extremely dense, lines of energy that extend vast distances into space. Despite their thinness, they have a significant mass and gravitational pull. Think of them as ultra-thin, yet extremely powerful threads woven into the fabric of the universe.
- Formation: Theoretical physicists propose that cosmic strings could have formed during the earliest moments after the Big Bang. As the universe rapidly expanded and cooled, it underwent several phase transitions, such as water turning into ice. These transitions may have left behind defects in the form of cosmic strings.
- Properties: Cosmic strings are believed to be narrower than a proton, yet their density is so high that a mile-long string can stretch as far as a mountain. Their gravitational effect is significant despite their thinness.
Cosmic strings and time travel
The idea that cosmic strings could facilitate time travel stems from their intense gravitational effects on space-time. Here’s how they could make time travel possible:
- Distorting space-time: According to general relativity, massive objects can distort the fabric of space-time. Because of their extreme density and mass, cosmic strings can cause significant distortions in the space-time around them.
- Closed time-like curves (CTCs): If two cosmic strings pass each other at high speeds or if one string forms a loop, the distortion of space-time can create closed time-like curves. CTCs are paths in space-time that loop back on themselves, theoretically allowing an object to return to its past. Traveling along such a curve could make backward time travel possible.
- Possible time travel mechanism: Theoretically, if a spacecraft can navigate the gravitational fields around two moving cosmic strings or a looped string, it may be able to follow the CTC and thus travel backward in time. However, this is purely speculative as we currently lack the technology to manipulate or detect cosmic strings.
Challenges and paradoxes
- Existence: Despite being a fascinating concept, cosmic strings have not yet been observed. They remain hypothetical constructs supported by certain theoretical models in cosmology and particle physics.
- Technological limitations: Even if cosmic strings exist, the technological requirements to interact with or manipulate them are far beyond our current capabilities. Harnessing their potential for time travel is purely theoretical at this stage.
- Paradoxes: The concept of traveling through time via cosmic strings introduces potential paradoxes, such as the famous “grandfather paradox,” where a time traveler could potentially alter past events in ways that preclude their own existence. Resolving these paradoxes is an ongoing challenge in the study of time travel.
4.Quantum Entanglement: Linking Moments in Time:
Quantum entanglement is one of the most intriguing and paradoxical phenomena in quantum mechanics. It occurs when two or more particles become intertwined in such a way that the state of one particle instantaneously affects the state of the other, no matter how far apart the particles are. This “spooky action at a distance,” as Albert Einstein famously referred to it, has been experimentally confirmed and has profound implications for our understanding of the universe.
The Basics of Quantum Entanglement
In quantum systems, particles such as electrons or photons can become entangled through various interactions. Once entangled, the properties of these particles become linked. For example, if you measure the spin of one entangled particle, you can instantaneously know the spin of the other, no matter how far apart they are. This instantaneous connection appears to violate the speed limit set by the speed of light, leading to fascinating discussions and experiments.
Connecting Moments in Time
Quantum entanglement challenges our classical understanding of time and causality. In classical physics, events are usually linked by cause and effect, with effects being transmitted at or less than the speed of light. However, entanglement suggests that particles can be correlated in ways that transcend normal space-time constraints.
Some physicists propose that entanglement could be used to transmit information across time, not just space. This idea stems from the fact that the quantum states of entangled particles remain linked even when they are separated by vast distances or time. If we could precisely manipulate these states, it might be possible to create correlations between events that are separated in time, effectively linking moments in time through quantum entanglement.
Theoretical implications and experiments
While the idea of using entanglement for time travel is still speculative, several theoretical models explore this possibility:
- Quantum communication across time: Researchers have proposed that information encoded in entangled particles could be retrieved at a different time, making a form of temporal communication possible. This concept relies on sophisticated quantum information protocols and precise control over quantum states.
- Temporal correlations: Some theories suggest that entanglement could create correlations between events occurring at different times, providing a framework for understanding the behavior of quantum systems over time. This could have implications for the development of new technologies in quantum computing and information processing.
- Quantum retrocausality: This controversial idea holds that future events can influence past events through quantum entanglement. Although it is still much debated, it challenges the traditional one-way flow of time and opens new avenues for exploring the nature of reality.
Challenges and Future Research
The practical application of quantum entanglement to connect moments in time faces significant challenges:
- Decoherence: Quantum states are extremely fragile and can be easily disrupted by their environment. Maintaining entangled states over long periods or distances is a major technical hurdle.
- Measurement and control: Entangled states are difficult to accurately measure and control. Any attempt to manipulate these states can collapse the entanglement, destroying the desired correlation.
- Theoretical validation: While interesting, many of these ideas are still theoretical and lack experimental validation. More research is needed to determine whether these concepts can be applied practically.
5.Closed Timelike Curves: Loops in Time:
A closed time-like curve (CTC) is a theoretical concept in the field of general relativity where an object’s world line (its path through space and time) curves back on itself, allowing the object to return to its past. This idea suggests the possibility of traveling backward in time, which raises fascinating but complex questions and paradoxes.
The Basics of Closed Time-Like Curves
- World line: In relativity, a world line is the path an object takes through the four-dimensional space-time continuum. Generally, these paths move forward in time.
- Closed curve: A CTC is a world line that curves back to its starting point, meaning that an object can, in principle, reach the point in space-time where it was before.
Theoretical basis
CTCs arise from solutions of the equations of general relativity, which describe how matter and energy distort space-time. Some specific solutions suggest that under certain conditions, space-time can be curved in such a way that these closed loops in time are possible. Here are some scenarios where CTCs can occur:
- Rotating black holes (Kerr black holes): According to general relativity, the space-time around a rotating black hole can be curved in such a way that it can allow for CTCs.
- Gödel Universe: Proposed by Kurt Gödel in 1949, it is a solution to the equations of general relativity that describes a rotating universe. In such a universe, the existence of CTCs is possible.
- Wormholes: Hypothetical tunnels connecting distant points in space-time can also allow for CTCs if they can be stabilized and manipulated in certain ways.
Paradoxes and Challenges
The existence of CTCs brings up several paradoxes and challenges that have puzzled scientists and philosophers:
- Grandfather Paradox: This is the most famous paradox. If you travel backward in time and prevent your grandparents from meeting, this would prevent your existence, which raises the question of how you could go back in time in the first place.
- Consistency paradox: This deals with actions taken in the past that could potentially change the timeline in a way that contradicts the original history. For example, if you travel backward and change a key event, what would happen to the future you came from?
- Self-consistency principle: Proposed by physicist Igor Novikov, this principle states that any action taken by a time traveler is always part of history, which ensures consistency and avoids paradoxes. Essentially, this means that you cannot change the past because your actions were always part of the historical timeline.
Current scientific view
While CTCs are mathematically possible within the framework of general relativity, their physical reality remains highly speculative:
1 . Energy requirements: Exotic matter with negative energy density may be required to create a CTC, something that has not been observed in the required
2. quantities. Quantum gravity: Many physicists believe that a full theory of quantum gravity (which would unify general relativity and quantum mechanics) could prohibit CTCs or resolve the paradoxes they present. However, such a theory remains elusive.
6.Tachyons: Hypothetical Particles of Faster-than-Light Travel:
Tachyons are a fascinating concept in theoretical physics, proposed as particles that travel faster than light. The name “tachyon” comes from the Greek word “tachys,” meaning “fast” or “rapid.” Despite being entirely hypothetical and yet to be observed in any experiments, tachyons present interesting possibilities and important implications for our understanding of the universe, especially in the context of time travel.
Theoretical background
The idea of tachyons arises from the special theory of relativity, formulated by Albert Einstein. According to this theory, nothing can travel faster than the speed of light in a vacuum, which is about 299,792 kilometers per second (186,282 miles per second). This speed limit is due to the relationship between energy, mass, and the speed of light: as an object approaches the speed of light, its mass effectively becomes infinite, requiring infinite energy to continue moving.
However, in the 1960s, physicists Gerald Feinberg and Arnold Sommerfeld explored the implications of hypothetical particles that could always travel faster than light. These particles were named “tachyons.” Unlike normal particles, which require more energy to move faster, tachyons require more energy to slow down, and as they lose energy, they get faster.
Properties of tachyons
Tachyons have several unique and paradoxical properties:
- Faster-than-light speed: By definition, tachyons move faster than light. This superluminal speed leads to various peculiarities in their behavior and theoretical implications.
- Imaginary mass: In mathematical terms, tachyons are described as having “imaginary mass.” This does not mean that they are figments of the imagination, but rather that their mass consists of complex numbers, leading to unusual physical properties.
- Energy relation: For tachyons, the relation between energy and velocity is inverted compared to normal particles. As they lose energy, they accelerate, potentially moving infinitely fast as their energy approaches zero.
Implications for time travel
The existence of tachyons would have profound implications for the concept of time travel. Special relativity suggests that if something can move faster than light, it can potentially travel backward in time, creating the possibility of time loops and causality violations. This notion is often represented by the famous “tachyonic antitelephone” thought experiment, where a message sent by a tachyon could theoretically be received before it was sent, creating a paradox.
Challenges and controversies
Despite their fascinating properties, tachyons face significant challenges:
- Lack of experimental evidence: To date, no experimental evidence supports the existence of tachyons. Particle accelerators and other detection methods have not yet observed these elusive particles.
- Causality paradoxes: The theoretical possibility of backward time travel and causality violation give rise to paradoxes that are difficult to resolve. These paradoxes challenge our understanding of cause and effect and the fundamental structure of space-time.
- Stability issues: Some theories suggest that if tachyons existed, they could cause instabilities in the vacuum of space, leading to catastrophic events. This potential instability raises further questions about the feasibility of their existence.
Current research and theoretical explorations
While tachyons remain a theoretical construct, they continue to inspire research and exploration in physics. Scientists study tachyonic fields in quantum field theory, string theory, and other advanced areas of theoretical physics to better understand their implications. These studies often provide deep insights into the nature of space, time, and the fundamental forces of the universe.
7.Time Crystals: Breaking Temporal Symmetry:
Introduction to Time Crystals
Time crystals are a fascinating and relatively new state of matter, first proposed by Nobel laureate Frank Wilczek in 2012 and experimentally realized in 2016. Unlike ordinary crystals, whose structure is repeated in space, time crystals exhibit a structure that is repeated in time. This means that their atomic configuration changes in a periodic manner not only in spatial dimensions but also in the temporal dimension.
Understanding Temporal Symmetry
In classical physics, symmetry plays a key role in understanding the properties of substances. Spatial symmetry involves patterns that are repeated at regular intervals in space, such as the repeating lattice structure of a diamond. On the other hand, temporal symmetry refers to patterns that are repeated over time.
Ordinary crystals break spatial symmetry because their atoms are arranged in a periodic lattice structure rather than being uniformly distributed. Time crystals break temporal symmetry because their atomic structure repeats at regular time intervals rather than being static or in thermal equilibrium.
How Time Crystals Work
The main feature of a time crystal is its ability to oscillate indefinitely without energy input, a property that appears to defy the second law of thermodynamics, which states that systems evolve toward thermal equilibrium. Time crystals achieve this by existing in a non-equilibrium state, which is maintained through periodic driving, such as the application of a laser or microwave pulse.
In a time crystal, atoms or spins oscillate between different states in a predictable, repetitive pattern over time. This oscillation is not caused by thermal motion or an external force but arises from the intrinsic properties of the system.
Experimental Realization of Time Crystals
The first experimental construction of time crystals was achieved using ions and nitrogen-vacancy centers trapped in diamonds. In these experiments, researchers applied periodic pulses to these systems, causing their components to oscillate in such a way that temporal symmetry was broken. The systems exhibited a stable, repeating pattern over time, confirming the existence of time crystals.
Potential applications and implications
While time crystals are still primarily the subject of theoretical and experimental research, their discovery opens up exciting possibilities for future applications, including:
- Quantum computing: Time crystals could serve as robust qubits for quantum computers, providing stability and coherence over long periods.
- Precision measurement: The stable oscillatory behavior of time crystals could lead to advances in precision timekeeping and measurement devices.
- New phases of matter: The study of time crystals could deepen our understanding of non-equilibrium phases of matter and lead to the discovery of new states of matter with unique properties.
Conclusion: The Journey Continues:
The quest to unravel the mysteries of time travel is a fascinating and continuing journey that combines science fiction dreams with cutting-edge scientific research. Although we have not yet broken the code to build a working time machine or discovered a practical way to traverse time, the discoveries and theories we have discovered demonstrate the incredible progress and potential in this field.
Each of the seven mind-blowing discoveries we have discussed – Einstein’s theory of relativity, wormholes, cosmic strings, quantum entanglement, closed timelike curves, tachyons, and time crystals – offers a unique perspective on how time can be manipulated or traveled through. These ideas push the boundaries of our understanding and challenge us to think beyond the limits of current technology and knowledge.
The journey to understand time travel is marked by both remarkable successes and persistent challenges. Theoretical physics provides us with frameworks and possibilities, but translating these into practical, testable, and observable phenomena remains a significant hurdle. For example, while time dilation has been experimentally confirmed, the creation or discovery of stable wormholes or cosmic strings remains entirely theoretical. Similarly, quantum entanglement and time crystals open doors to new realms of possibility, yet their practical applications in time travel are still speculative and require much more research.
As we continue to delve into the mysteries of time, each discovery takes us one step closer to understanding its true nature and potential. This constant exploration fuels our imagination and scientific curiosity, keeping the dream of time travel alive. The journey is not over yet; in fact, it has just begun. Future advancements in technology, deeper insights into the fabric of the universe, and breakthroughs in our understanding of quantum mechanics and general relativity could all play a key role in making time travel a reality.
The journey towards unlocking time travel is a testament to human ingenuity and the constant pursuit of knowledge. As we stand on the verge of discoveries, it is clear that the exploration of time and its mysteries will continue to fascinate and inspire us. The future holds endless possibilities, and with each step, we get closer to realizing the dream of time travel.
Stay tuned and connected with the latest developments in theoretical physics, because you never know when the next important discovery will reshape our understanding of time and the universe. The journey continues, and it promises to be an exciting adventure full of surprises, challenges, and unprecedented discoveries.